The effect of preconditioning on liver regeneration after hepatic resection in cirrhotic rats
Article information
Abstract
Background/Aims
Ischemic preconditioning (IP) decreases severity of liver necrosis and has anti-apoptotic effects in previous studies using liver regeneration in normal rats. This study assessed the effect of IP on liver regeneration after hepatic resection in cirrhotic rats.
Methods
To induce liver cirrhosis, thioacetamide (300 mg/kg) was injected intraperitoneally into Sprague-Dawley rats twice per week for 16 weeks. Animals were divided into four groups: non-clamping (NC), total clamping (TC), IP, and intermittent clamping (IC). Ischemic injury was induced by clamping the left portal pedicle including the portal vein and hepatic artery. Liver enzymes alanine transaminase (ALT) and aspartate aminotransferase (AST) were measured to assess liver damage. Terminal deoxynucleotidyl transferase-mediated deoxyuridine triphosphate nick-end labeling (TUNEL) staining for apoptosis and proliferating cell nuclear antigen (PCNA) staining for cell replication were also performed.
Results
Day-1 ALT and AST were highest in IP, however, levels in NC and IC were comparably low on days 1-7. There was no significant correlation of AST or ALT with experimental groups (P=0.615 and P=0.186). On TUNEL, numbers of apoptotic cells at 100× magnification (cells/field) were 31.8±24.2 in NC, 69.0±72.3 in TC, 80.2±63.1 in IP, and 21.2±20.8 in IC (P<0.05). When regeneration capacity was assessed by PCNA staining, PCNA-positive cells (cells/field) at 400× were 3.4±6.0 in NC, 16.9±69 in TC, 17.0±7.8 in IP and 7.4±7.6 in IC (P<0.05).
Conclusions
Although regeneration capacity in IP is higher than IC, the liver is vulnerable to ischemic damage in cirrhotic rats. Careful consideration is needed in applying IP in the clinical setting.
INTRODUCTION
Hepatic dysfunction due to ischemic injury by hemorrhage or prolonged interruptions of blood supply during liver resection and liver transplantation have been continuing problems in the past few years.1 Particularly, blocking of hepatic blood flow that is occasionally applied to prevent massive hemorrhage in liver surgery artificially induces hepatic ischemic injury. Oxidative stress and cell damage, which are induced by the excess production of oxygen radicals due to activation of Kupffer cells, occur within a few minutes during reperfusion.2-5 In addition, the respiratory chain involved in ATP synthesis in mitochondria of hepatocytes and sinusoidal endothelial cells is markedly reduced due to the re-influx of oxygen.4 Although several agents have been introduced to prevent ischemic injury, their beneficial effects have not been proven.6 With the increase in hepatectomy and liver transplant, various methods have been investigated to reduce ischemic injury of liver tissue at the time of reperfusion after blocking blood flow.7,8
One method reported to reduce injury is ischemic preconditioning (IP), in which blood flow is blocked for a short time initially with recovery of blood flow by reperfusion, subsequently allowing blood flow to be blocked for a relatively long period.9 In IP, nitrogen oxide synthesis produces enzymes that inactivate caspases.10 The suppression of caspases prevents apoptosis of liver cells.11,12 Ischemia during the short period of IP directly prevents damage to the hepatic parenchyma. While ischemic damage is progressing, diverse cytokines are secreted,13 and the development of oxidative stress is reduced.14 By exposing to the ischemic environment for a short period, IP generates a protective environment. IP also promotes liver regeneration via the adenosine A2 receptor pathway in Kupffer cells.15 Although studies of liver regeneration in normal rats after IP have been already performed, results on liver cirrhosis patients encountered in the clinical setting are not abundant.
Therefore, this study examined the difference in liver regeneration capacity after ischemic injury using the liver cirrhosis animal model established previously.16 In addition, since most patients with indication for surgical treatments are associated with liver cirrhosis, the possibility of the clinical application of IP for liver regeneration was examined in liver cirrhosis animal models.
MATERIALS AND METHODS
The animal model
Experimental animals were maintained in a pyogen-free (SPF) animal facility at the Clinical Research Center, Yonsei University College of Medicine, under constant room temperature (22℃) and humidity (55%), with a supply of sterile drinking water and sterile animal feed according to the standards of the Association of Assessment and Accreditation of Laboratory Animal Care International. In addition, animal experiments were conducted after approval of the experiment procedures by the ethical animal experiment guideline established by the Department of Experimental Animals, Clinical Medical Research Center, Yonsei University College of Medicine.
Induction of liver cirrhosis
Liver cirrhosis was induced by injecting 300 mg/kg thioacetamide (Sigma, St. Louis, USA) intraperitoneally into 8 week-old Sprague-Dawley (SD) white rats, twice a week, for 16 weeks.16
Determination of the ischemic period
To compare the extent of liver damage according to the ischemic period, the left portal pedicle including hepatic artery and portal vein was clamped for 30, 60, 90, or 120 min, and liver tissue was obtained from the ischemic area. The degree of apoptosis was assessed by the ApopTag Peroxidase in situ apoptosis detection kit (S7100; Chemicon International, Temecula, CA, USA). Briefly, the tissues were deparaffinized with xylene, followed by 100%, 75%, and 50% ethanol sequentially, and washed one time with phosphate buffered saline (PBS) for 5 min. The samples were treated with Proteinase K (20 µg/mL, DAKO proteinase K Cat NO. S3020) for 15 min at room temperature, and rinsed with distilled water twice prior to incubation with 3% hydrogen peroxidase for 5 min at room temperature and washing with PBS. After removing moisture from around the tissue, equilibration buffer was sprayed to the tissues, and incubated for 10 min at room temperature. Working strength TdT enzyme (40 µL) was added and the samples incubated at 37℃ for 1 hour. They were then treated with STOP/WASH buffer, shaken for 15 seconds, and incubated at room temperature for 10 min. After washing with PBS, anti-digoxigenin conjugate was added to the slides, and incubated at room temperature for 30 min. After washing with PBS, the samples were treated with the peroxidase substrate diethyl aminobenzidine. After washing three times with sterile water for 1 min each, the sample was incubated in sterile distilled water for 5 min at room temperature. Counter staining was performed by adding hematoxylin for 5 min, process washing, and then treating with 1% EtOH+HCl twice, followed by washing with ammonia solution 10 times.
Study design
Experiment groups
Liver cirrhosis-induced Sprague-Dawley white rats (N=12) were equally (N=3) divided into the non-clamping (NC), total clamping (TC), IP, and the intermittent clamping (IC) groups (Fig. 1).
Induction of ischemia and hepatectomy
Zoletil (30 mg/kg, Virbac, Carros, France) was intraperitoneally injected and after the animal was fully sedated, the abdomen was opened through a midline skin incision and the liver hilum was exposed. The left portal pedicle was identified and ischemia was induced by blocking blood flow through clipping the portal vein and hepatic artery running into the left hepatic lobe using aneurysmal clips. Immediately after the induction of ischemic injury or 90 min after laparotomy in the NC group, the total injured liver, approximately 75% of the area of the total liver was resected.
Liver function test
Immediately prior to ischemic injury (day 0) as well as 1, 3, 5, and 7 days after injury, rats were anesthetized and blood was collected from the tail vein. The blood was centrifuged at 3,000 rpm for 15 min, and using the supernatant, serum aspartate aminotransferase (AST) and alanine aminotransferase (ALT) were measured using a biochemical analyzer at the Korean Animal Clinical Research Center.
Evaluation of liver cirrhosis
In each experimental group, the presence or absence of liver cirrhosis was evaluated by trichrome staining (HT15 Trichrome Stain [Masson] Kit, Sigma, St. Louis, USA) as follows. The deparaffinized slides were hydrated, incubated with heated Bouin's solution (56℃) for 15 min, and cooled by immersing in water. They were washed until the yellow color disappeared from the tissues, and stained with Weigert's Iron Hematoxylin solution for 5 min. Slides were washed with running water for 5 min and immersed in distilled water for a while followed by staining with Biebrich Scalet-Acid fuchsin for 5 min, and incubation in phosphotungstic/phosphomolibdic acid solution for 5 min. They we then incubated in aniline blue solution for 5 min, incubated with 1% acetic acid for 2 min, washed, and dehydrated with alcohol, and then collagen fibrosis was examined.
Evaluation of hepatocyte injury caused by liver ischemia
To evaluate the level of hepatocyte injury caused by liver ischemia in the experimental groups, hematoxylin and eosin (H&E) staining and terminal deoxynucleotidyl transferase-mediated deoxyuridine triphosphate nick-end labeling (TUNEL) assay were performed as follows.
H&E staining
Liver tissues were fixed with 10% formaldehyde and prepared as paraffin slides. After the deparaffinization procedure of treating sequentially with xylene, and then 100%, 75%, and 50% ethanol; hematoxylin staining, washing, eosin staining, washing, dehydration with lower concentration of alcohol up to high concentration, and xylene treatment were performed sequentially, and the injury level of liver tissues was assessed by hyalinization.
Comparison of the level of liver injury by TUNEL assay
The level of liver injury in the groups was compared by extracting the liver immediately after ischemic injury induction and performing TUNEL assay. In brief, after sequential deparaffinization and washing with PBS, the slides were incubated with proteinase K for 15 min, treated with 3% hydrogen peroxide for 15 min, and washed with PBS. The slides were pretreated with the equilibration buffer (19054; QIAGEN, USA) for 20 min and reacted with the TdT mixture for 1 hour in a 37℃ humidified chamber. Samples were then treated with the stop/wash buffer for 10 min, and washed with PBS. Antidigoxigenin conjugated solution at room temperature was added for 30 min, washed with PBS, followed by reaction with diethyl aminobenzidine (00-2014; Invitrogen, San Francisco, CA, USA) for 5 min. Subsequently, apoptosis was examined.
Comparison of regeneration capacity of the liver after ischemic injury
To evaluate the cell division capacity, proliferating cell nuclear antigen (PCNA) staining was performed as follows: Liver extracted at the time of sacrifice of cirrhosis rat model was added to 10% formalin solution, and embedded in paraffin by conventional methods. After the hydration procedure with Xylene and alcohol, the slides were incubated with anti-PCNA antibody diluted to 1:50 at 4℃ for 24 hours, washed with PBS, and then incubated with anti-mouse immunoglobulin G antibody (Sigma M9902) diluted to 1:100 at room temperature for 40 min. They were then incubated with the PAP Complex (Sigma P2416, in mouse) diluted to 1:100 at room temperature for 40 min, and stained with 0.025% 3,3-diaminobenzidine in PBS and 0.045 % hydrogen peroxide at room temperature for 3 min, and counterstained with hematoxylin. PCNA showing immune response was extracted by the hydrogen peroxide method, and examined under a light microscope. After counting 25 fields, the averages were obtained, and statistical analysis was performed. After PCNA staining, PCNA-positive cells were counted in a 10-µm × 10-µm area. At 400× magnification, 25 areas were selected randomly, and the number of stained cells according to each staining method was counted (cells/field).
Statistics
Using the statistics program, SPSS 15.0 (SPSS, Chicago, IL, USA), differences in apoptosis and cell division capacity among the experimental groups were assessed by analysis of variance. The results were presented as mean±standard error of the mean, and cases with P<0.05 were considered statistically significant.
RESULTS
Determination of ischemic injury period by the comparison of the level of apoptosis
After 60 min of ischemia, the degree of injury was not significant as evaluated by assessing for apoptotic cells in areas of Zone I. After 90 min, the extent of the injured area in Zone I had expanded; and after 120 min, most areas of the liver tissue were severely injured (Fig. 2). Hence, 90 min was selected as the ischemic period for the experimental groups since this induced the most appropriate level of liver injury.
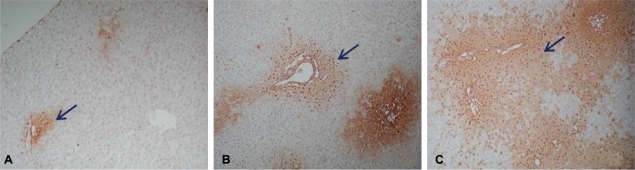
Degree of apoptosis of the liver tussue from the rats after ischemic injury assessed by ApopTag peroxidase in situ apoptosis detection kit (×40). The brownish spots indicated by arrows represent apoptotic cells. (A) 60-min ischemic injury, (B) 90-min ischemic injury, and (C) 120-min ischemic injury.
Levels of serologic enzymes (AST, ALT) for hepatic function
On day 1, ALT and AST were markedly increased in the TC and IP groups with the highest level of both AST and ALT in the IP group on day 1, and returned to basal levels on day 3 and 5 after ischemic liver injury (Fig. 3). In the NC and IC groups, AST and ALT were comparably low for 7 days. There was no significant correlation of AST or ALT level with experimental groups (P=0.615 and P=0.186, respectively).
Comparison of the degree of hepatocyte injury
Following the induction of ischemia according to the various methods, liver tissue obtained from the ischemic injury area was stained with H&E, and the degree of tissue injury was examined (Fig. 4A-D). The level of liver fibrosis was compared through trichrome staining (Fig. 4E-H) and the level of apoptosis was compared using the TUNEL assay (Fig. 4I-L). In the TUNEL assay performed to evaluate the ischemic injury of liver, the numbers of apoptotic cells at 100× magnification were 31.8±24.2 cells/field in NC group, 69.0±72.3 in the TC group, 80.2±63.1 in the IP group, and 21.2±20.8 cells/field in the IC group. Compared to the control, significant ischemic injury of the liver was detected in the TC and IP groups (P<0.05). In the NC group, liver cirrhosis had progressed and associated with liver fibrosis (Fig. 4A and E). In the rest of the experimental groups, liver fibrosis was severe compared to the NC group (Fig. 4F-H).

H&E (A-D), trichrome (E-H), and TUNEL (I-L) stains of the liver after various ischemic injuries (×40). The arrow identifies the thick blue bundles of separating collagen (A, E, and I: non-clamp [NC]; B, F, and J: total clamp [TC]; C, G, and K: ischemic precondition [IP]; D, H, and L: intermittent clamp [IC]).
Comparison of the liver regeneration capacity
To assess the effect of ischemia on liver generation capacity in each group, rats were sacrificed 7 days after hepatectomy, and the residual liver was stained with H&E (Fig. 5A-D), trichrome (Fig. 5E-H), and PCNA (Fig. 5I-L). Liver regeneration capacity was better in the IP group (Fig. 5C, G, and K) than the TC (Fig. 5B, F, and J) or IC groups (Fig. 5D, H, and L) and was even comparable to the NC group (Fig. 5A, E, and I). When liver regeneration capacity was assessed by PCNA staining, the number of PCNA stained cells at 400× magnification was 3.4±6.0 cells/field in the NC group, 16.9±69 in the TC group, 17.0±7.8 in the IP group, and 7.4±7.6 cells/field in the IC group. Compared to the control group, the regeneration capacity of the residual liver in the TC and IP groups was higher (P<0.05, Fig. 6)

H&E (A-D), trichrome (E-H), and proliferating cell nuclear antigen (PCNA) stains (I-L), (×100) at 7 days after various kinds of ischemic injury (A, E, and I: non clamp [NC]; B, F, and J: total clamp [TC]; C, G, and K: ischemic preconditioning [IP]; D, H, and L: intermittent clamp [IC]).
DISCUSSION
With accumulated experience with hepatectomy and liver transplantation, as well as the development of new techniques, indications for the procedures have been increasing. However, apoptosis of liver cells occurring during hepatectomy and liver transplant may be detrimental for patients, thus prompting recent active studies on the apoptosis occurring during liver ischemia and reperfusion.11,17 Several methods have been suggested to decrease apoptosis. However, prior to clinical application of the methods, their effectiveness needs be proven in experimental animal models first.18
Ischemic injury was induced by clipping the portal vein and hepatic artery entering the left lobe of the liver. In the total ischemic model, severe injury to the liver tissue and hepatocyte apoptosis was observed. During ischemia, leukocytes increase, macrophages are activated, and the injury of liver tissues and hepatocytes is mediated by the interaction of the inflammatory reaction, free radical production, and apoptosis. These mechanisms lead to injury at the time of reperfusion following ischemia, and the disruption mitochondrial ion homeostasis by hepatocyte ischemia causes cellular calcium influx resulting in protein and DNA damage. Xanthine oxidase synthesis, which generates free radicals, is also accelerated.19,20 In addition to free radical overproduction through xanthine oxidase synthesis, local vascular constriction is induced by the inflammatory reaction exacerbating cellular ischemia and edema.21 The apoptosis of hepatocytes during reperfusion is characterized by cell and organelle edema,22 with resultant expulsion of cellular contents and karyolysis.23 Because of the deterioration of the respiratory chain in mitochondria due to oxygen influx during reperfusion, ATP synthesis is disturbed, and apoptosis occurs due to oxidative stress. The increase in reactive oxygen species during reperfusion induces the disruption of the hepatocyte membranes and the signal transduction system as well as destruction of red blood cells. This consequently induces deterioration of hepatic function and liver damages.24
It has been reported that IP can be applied to prevent liver injury, and that the activation of Kupffer cells was lower than TC, with the major effect of protecting hepatocytes and sinusoidal cells from apoptosis.25 It is considered that the IP procedure protects liver tissues and liver cells by exposing them to the ischemic environment for a short period. Ischemic treatment methods are as follows: 1) total ischemic treatment where ischemia occurs only during liver surgery; 2) IP treatment that induces ischemia after ischemia/reperfusion; and 3) the intermittent ischemic treatment that repeats ischemia and reperfusion.
In total ischemic treatment that blocks blood flow completely, the level of liver injury is severe, and liver regeneration is difficult after surgical treatments. In intermittent treatment, repeated ischemia and reperfusion is burdensome, and during reperfusion, hemorrhage may be induced at the resected surface. In comparison, IP treatment induces relatively less liver injury, and may result in more effective liver regeneration after surgery. Ischemic treatment during liver transplant or liver resection may cause fatal injury to liver tissue, and thus when surgical treatments are performed, more effective methods should be developed to minimize liver damage, and promote regeneration.
Cytokines and growth factors expressed during liver regeneration are associated with expression of their receptors. It has been reported that during liver resection or injury, epithelial-cell growth factor receptor and the hepatocyte growth factor receptor c-Met are activated.26,27 Among these, the endogenous factor, heat-shock protein-70 has been shown to have the function of protecting cells and tissues during injury. Based on previous reports, heat-shock protein-70 is overexpressed during IP treatment and thus activates various signal transduction systems pertinent to regeneration.28
Our study performed the IP procedure that has been used to reduce ischemic injury, and the level of liver injury and regeneration capacity were compared to other procedures blocking blood flow. Particularly, since most liver transplants are associated with liver cirrhosis, animal models should also have similar clinical features. Generally, for the induction of liver cirrhosis in rats, thioacetamide, carbon tetrachloride, dimethylnitrosamine, and other drugs are used. Carbon tetrachloride needs to be diluted in oil, and thus intraperitoneal administration is difficult. In addition, it may induce pain in rats, and thus is not efficient.16 The survival rate of rats treated with dimethylnitrosamine is very low, and thus this method is also not suitable. However, thioacetamide has advantages: it could be administered into the peritoneal cavity, and it induces liver cirrhosis that is closest to human liver cirrhosis.16 Therefore, in our study, liver cirrhosis was induced in Sprague-Dawley rats using intraperitoneal administration of thioacetamide.
First, to determine the ischemic period, the level of apoptosis according to various periods were compared. If the injury is too small, it would be difficult to distinguish the level of injury from the regeneration capacity. On the other hand, if injury is too severe, the regeneration capacity would noticeably deteriorate. Hence, in our study, 90 min was selected as the optimal ischemic period. After ischemia, the level of liver-cell damage was most severe in TC group. The IP group also developed injury; nonetheless, comparison of the regeneration of hepatocytes shows that the regeneration capacity of IP group was good. This could be explained by the fact that in liver damage, cell proliferation is an indicator of liver regeneration, and in IP treatment, increased resistance by the suppression of c-fos gene during ischemia and reperfusion could contribute to reduced injury.29,30 In addition, it is thought that IP is also associated with the above-mentioned heat-shock protein-70 mechanism improving the regeneration capacity of cells.28 In other words, it is thought that in hepatectomy for cases associated with liver cirrhosis, the application of IP procedure may improve the regeneration capacity of liver after surgical treatments.28 This study confirms that the liver regeneration capacity in the IP group is high. Nonetheless, during the treatment, the level of ischemic injury was very severe almost to the level of the TC group, and thus it appears that for application of IP procedure to cases associated with liver cirrhosis, more comprehensive attention is required.
Acknowledgements
This study was supported by a grant of the Korea Healthcare technology R&D Project, Ministry for Health, Welfare & Family Affairs, Republic of Korea (A084120).
Abbreviations
ALT
alanine aminotransferase
AST
aspartate aminotransferase
H&E
hematoxylin and eosin
IC
intermittent clamping
IP
ischemic preconditioning
NC
non-clamping
PBS
phosphate buffered saline
PCNA
proliferating cell nuclear antigen
TC
total clamping
TUNEL
terminal deoxynucleotidyl transferase-mediated deoxyuridine triphosphate nick-end labeling